Colloid and
Surface Science Aspects of Disinfectants
Garret Lau
Carla Ng
Pintu Saha
University at Buffalo, Department of
Chemical Engineering
Disinfectants represent a wide range of substances that are used in various applications. The food industry requires the use of disinfectants to sanitize food preparation areas, and serve preservative functions. Chlorine and other organic oxidizers are employed for the purification of drinking water. Hospitals and clinics rely on disinfectants to sanitize their medical facilities. Disinfectants are even used for their preservative abilities in paints, inks, cosmetics, and other industries. And of course, the disinfectants most people are familiar with, household disinfectants, serve to help us with controlling germ and bacteria levels in our kitchens, bathrooms, and bodies. Despite the variety in disinfectant materials, they all strive for one desired characteristic: selective toxicity. Disinfectants are engineered to kill bacteria, viruses, and mildew, yet be safe to possible human contact.
In the United States, the primary regulations that disinfectants must abide by are established by the Environmental Protection Agency (EPA) and the Food and Drug Administration (FDA). The EPA regulates drinking water purification, finding ways to limit or replace chlorine as a disinfectant, fearing the biological contamination of organic chlorinated byproducts (w4). Household disinfectants found in soaps and other cleaners are regulated by the FDA, where products are tested and examined for public health and safety (w6). While many disinfectants pass through government agencies for safety approval, we chose to focus our scope on common household disinfectants.
Household disinfectants must meet several criteria. Besides having anti-microbial properties and being safe to use around humans and the environment, household disinfectants are almost exclusively incorporated into a cleaner that provides multipurpose capabilities, aside from just killing germs. A 1996 Hard Surface Cleaner Market Study determined that consumers desire cleaners that disinfect, cut grease, deodorize, and at the same time be cost effective (14). Bathroom cleaners were expected to remove soap scum and stains while leaving no film or residue (14). Cleaners must possess a dispersive quality – the ability to be sprayed or spread onto a surface for the necessary time required for disinfection and cleaning. This often means the incorporation of surfactants and the use of colloidal properties. Soaps and detergents frequently contain moisturizers to alleviate the harsh conditions on the skin. Despite these many considerations in household cleaners, disinfectant and antibacterial properties are among the most important aspects of cleaners consumers use most often.
While consumers worry about the sterilization capability of cleaners, a distinction must be made between the degrees of sterilization. Many common household cleaners are simply antibacterial. Hand soaps and liquid dish detergents contain triclosan, also known by its trade name Microban, which is strictly an antibacterial (5). Salmonella, E. coli, and bacteria that cause strep throat and staph infections are typically the common targets of triclosan. A true disinfectant also kills viruses and other pathogens along with bacteria. Alcohols, bleach (sodium hypochlorite), quaternary ammonium compounds, and certain oils comprise the most familiar household disinfectants. All disinfectants used in household cleaners must meet EPA or FDA approval. Regulations outlined by the EPA include stringent tests against Salmonella choleraesuis, Staphylococcus aureus and other bacteria, along with a performance mandate that in order to be termed a disinfectant for use on hard materials, the disinfectant must kill on 59 out of each set of 60 carriers and is required to provide effectiveness at the 95% confidence level (w5).
The increasing popularity of disinfectants in common household products is a recent trend. Between 1997 and 1999, manufacturers introduced 700 everyday products claiming antibacterial or disinfectant properties (5). Disinfectant cleaners make up half of the $2.1 billion cleanser market in the United States (15). Marketing household disinfectants is not about convincing consumers to sterilize their homes – studies show consumers already have this fear. Rather, the effectiveness of the cleaner in cleaning and disinfecting as compared to other brands is important, along with its relative price compared to other brands. Brand name cleaners may not necessarily provide a better product – often generic brands contain the same concentration of active ingredients.
Final product considerations reside in packaging and sales factors. Typical cleaners sell for $2-$4 for 32 ounces, representing the smaller sample size for household use (5). Packaging of disinfectants mostly involves the chemically inert plastic bottles that cleaners are supplied in. Variations exist in the aluminum spray cans for aerosol cleaners, and now technology is finding ways to introduce disinfectants into other mediums. N-halamine structures have been polymerized with a grafting process into cellulose containing and nylon fabrics, giving everyday materials anti-microbial properties within themselves (16). Triclosan is also being incorporated into a polymer for use in fabric seat covers, tables, chairs, and clothing sanitation (9). With so many methods of incorporating disinfectants into the household and the relative inexpensiveness of processing these materials, there seems no immediate decline to the increasing market of disinfectant products.
Components and Compositions of
Disinfectants for Household Use
Disinfectants for household use are divided into four main subcategories, as follows: alcohol, chlorine compounds, iodine compounds and quaternary ammonium compounds. These disinfectants are active at many pH levels. Iodines, for example, are most effective in the lower pH ranges, from ~2-6, whereas chlorines work best at the higher end, from 6-10. Quatrenary ammonium compounds are the most versatile, with a working range between 3 and 10.5 (2, Fig 23.1, p.477). For this reason, household products containing disinfectants are similarly solutions at various pH levels.
One of the most common chemicals present in the average person’s medicine cabinet is rubbing alcohol. It is often used as an antiseptic to clean minor wounds, and also as a hard surface cleaner. Like many chemical disinfectants, alcohols are generally considered to be nonspecific antimicrobials. They show a multiplicity of toxic effect mechanisms. This has important implications for the spectrum, speed and overall effectiveness of alcohol as a disinfectant. Not all alcohols show bactericidal effect; the amount of inhibition increases with the chain length of the alcohol (see 10, Table1, p.800).
Chlorine compounds are some of the most active ingredients in disinfectants. Use of chlorinated lime as a deodorant for sewage goes as far back as 1854 in Great Britain. Calcium hypochlorite has now largely replaced the older chlorinated lime, and sodium hypochlorite is th emost active principle of many household products. Various types of algae, bacteria, fungi, protozoa and viruses have shown resistance to hypochlorites (2, Table 7.1, p.143). The bactericidal action of hypochlorites is caused by the release of hypochlorous acid and contributions of hypochlorite ions (OCl-). Hypochlorites are subject to gradual deterioration over a period of time, which depends on three main factors. The most important factors are the pH and temperature of the environment. The lower the pH, the less stable the solution, but the more germicidal its action.
Chlorine dioxide is used a great deal for drinking water and wastewater treatment (2). It has the ability to break down phenolic compounds and removes phenolic tastes and odors from water. There are numerous antimicrobial chlorine compounds, but a major advantage of this particular formulation is that is does not form trihalomethanes (THMs) or chlorophenols, which are both harmful to the environment and have been coming under scrutiny by environmentalists making groundwater studies.
The major advantages of chlorine compounds are that they have very fast reaction times and are effective biocides for a broad spectrum of microorganisms. They are inexpensive compounds that do not foam, are not temperature dependent, and can be used in liquid or powder form. The main disadvantages are that chlorine is unstable in concentrate. It reacts strongly with organic materials and is corrosive to metals. More importantly, it is unfriendly to the environment.
Iodine, the heaviest of the common
halogens (126.9 g.mol), melts at 113.5ºC to a black liquid, and is a valuable
ingreadient in antiseptics. Iodine is a
highly reactive substance combining with proteins partly by chemical reations
and adsorption. Iodine-based
disinfectants can be divided into three main groups according to the solvent
and substances interacting with the iodine species: pure aqueous solutions,
alcoholic solutions and iodophoric preparations. They exhibit essential differences in their chemical and
microbiocidal properties. The iodine
compounds not only kill microorganisms but also interact with the materials to
be disinfected. To understand these
interactions, knowledge about the particular species, solvent, equilibrium concentrations
and individual reactivity is essential.
Iodine ions are often added to increase the solubility of iodine in water. This increase takes place by the formation of triiodide, I3-. Pure aqueous solutions, for the iodine-water system, produce at least ten iodine species:
I-, I2, I3-, I5-, I6-, HOI, OI-, HI2O-, I2O2-, H2OI+, and IO3-.
The ratio of their formation depends on the concentration of iodine.
Iodophors are polymeric organic molecules, such as alcohols, amides and sugar, which are capable of forming iodine species. This results in reduced equilibrium concentrations of species compared with those of pure aqueous solutions with the same total iodine and iodide concentrations. Since iodophoric preparation always contains appreciable iodide, the relevant species tat must be considered are restricted to I-, I2 and I3-, for the following simplified reactions (2, p.168):
I2 + R ßà R.I2
I3- + RßàR.I3-
I-+ R ßàR.I-
R represents the structural regions of the iodophor molecule capable of forming complexes by electronic effects.
An important solubilizing agent and carrier for iodine is poly(vinylpoyrrolidinone) (PVP). PVP-iodine is externally used on humans as an antiseptic. Some commercial brands are Betadine and Isodine.
Quaternary ammonium compounds are
often used in contact lens solutions for cleaning and preservative purposes.
The antibacterial precursors of the quaternary ammonium compounds (“quats”) are aliphatic long-chain ammonium salts. The direct counter part of soap may be considered as a primary ammonium salt. Both are surface-active substances. In soap, the anion contributes the hydrophobic part and the primary ammonium salt (the cation) is hydrophobic.
The primary long-chain ammonium salts are derived from the weakly basic aliphatic amines. Their aqueous solutions require a pH low enough to counteract hydrolysis and partial liberation of the amine base. Because quats ae salt bases, they remain in solution in acidic as well as in basic media. Quaternary ammonium salts produce bacteriostasis in very high dilutions. This property is associated with the inhibition of certain bacterial enzymes, especially those involved in respiration and glycolysis.
Among the many quaternary ammonium salts available, only a small number are of interest as antibacterial agents. Among them are: benzalkonium chloride, alkylbenzyldimethylammonium chloride, methydimethyl ammonium chloride, methylbenzethonium chloride, hexadecylpyridinium chloride, and alkylisoquinolinium bromide (10).
It is evident from the varied examples of disinfectant chemicals above that we as consumers have a wide range of products to choose from when it comes to ridding our homes of germs. Each has specific properties, advantages and disadvantages and it is important to keep this in mind, as well as the intended use, when choosing an appropriate cleaning product.
Colloids
in Disinfectants
Colloid science is concerned with the study of materials that exist as dispersions in a medium of some other material. They are sometimes defined as particles that would remain suspended in water for an extended amount of time. A colloid differs from a true solution in that the dispersed particles are larger than normal molecules, though they are too small to be seen with a regular microscope. The typical size of a dispersed particle is from a few nanometers to several micrometers. The size of those particles ranges from about 1 x 103 pm to about 2 x 105 pm in size. The consequences of the small size include a high surface area so that the properties of the interfaces may become important. The common element among all the types of colloids is the fact that they are held in suspension by electrostatic interaction with water molecules. Another important parameter is the thermal motion, which dominates the dynamic properties. Crucial examples include, food, paint, and household products (12).
Colloids in which the continuous phase is water are classified as follows: hydrophilic colloids, hydrophobic colloids and association colloids. The first two types of differ from each other by their chemical configuration and/or composition.
Hydrophilic colloids are large molecules that contain functional groups as an integral part of their structure. The functional groups form hydrogen bonds with water molecules. Common examples of hydrophilic colloids are proteins and synthetic polymers. Two examples of hydrophilic colloids are depicted below (11):
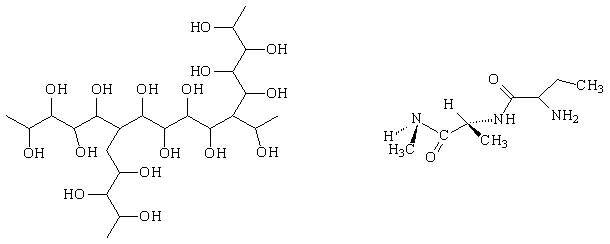
Figure 1: Hydrophilic colloids
Hydrophobic colloids are substances that have charged
surfaces in water, and form an electrical "double layer" that holds
them in suspension. Clays form a negative charge on their surface when placed
in water, and remain in suspension by the electrostatic interaction between the
negative surface charge and positive charges from cations in the water. The figure below depicts the colloidal clay
particles that are suspended in solution by electrostatic interaction (11).
Figure2:
Hydrophobic Colloid
The third type of colloids are "association" colloids.
These are molecules that have two parts to their molecular structure, a
hydrophobic part and a hydrophilic part. Soaps and detergents form association
colloids in water. Their molecular structure is similar to the illustration
below, where the carboxylic acid group is the hydrophilic portion and the
hydrocarbon chain is the hydrophobic part of the molecule (w8).
Figure3: 'association Colloid
Association colloids are the type that is used in the fabrication of disinfectants because of they possess some germicidal properties. They are also used in cationic detergents. Association colloids form self-assembly systems. Some examples are micelles, reverse micelles, vesicles, micro-emulsions and the monolayers and bilayers of some cell membranes. These monolayers and bilayers represent the building blocks of biomembranes (w2).
The word surfactant describes the activity of the molecule. They are SURFace ACTive AgeNTs; in other words a surfactant is a molecule that tends to align itself at a surface or interface. Surfactants fall into four broad categories: anionic, nonionic, cationic and amphoteric. They are described as being amphiphilic, in a sense that they have strong attraction towards both polar solvents (hydrophilic) and non-polar solvents (hydrophobic), and as a result they will concentrate at the interface between the two (11).
Surfactants are often portrayed as having a head and a tail. The head is said to be hydrophilic, it can either be ionic or non-ionic, and it is usually depicted as a circle. The tail is said to be hydrophobic and it is generally represented as a long hydrocarbon chain. The hydrocarbon is “water-hating” which thus means it is “oil-loving”. Normally surfactants lower the surface tension of water. Schematics commonly used to depict surfactants are shown below, along with the formula for an actual surfactant (11):
Figure 4: Anionic Surfactant
Surfactants are often characterized by their configurations. Sodium Oleate is an example of a molecule
that can be classified as surfactant.
It is a type of surfactants that is commonly used in the fabrication of
disinfectants. The oleate ion is
derived from triolein, (a triglyceride), by the hydrolization process. It consists of a glycerol with three ester
linkages. Overall, anionic surfactants
are the most used, often in conjunction with non-anionic ones, in order to yield
even greater stability in solutions.
Some
types of surfactants that are used in disinfectants are:
Sodium
palmitate
Sodium
myristate
Sodium
stearate
Note that the main difference between
these surfactants is the carbon chain length.
The implications of this will be discussed in greater detail in the next
section.
How
Colloids and Disinfectants Interact
There are certain characteristics that a disinfectant must possess in order to be effective. Soiled surfaces, from kitchen counters to bathroom sinks to skin often contain oily residues. Germs are trapped within these residues, where they are impervious to washing by water. The presence of organic matter may also impede the disinfectant itself. Two of the most commonly used disinfecting chemicals are chlorine and glutaraldehyde. While they have excellent germicidal properties, both are susceptible to inactivation by organic material (w10). Such organic materials may be part of the “dirt” itself that one is trying to clean off a surface, or it may be in the form of a biofilm, such as forms inside of toilets and septic systems.
In order to design an effective disinfectant, one must understand how the germicide will work in the environment where it will be used. This means that the interaction between each component of the cleansing agent and the “target” must be understood. Although the mechanism of germicide action has not been extensively studied, most scientists hypothesize that the interaction of the chemical agent with the cell membrane of the microbial species is key. The following is one possible sequence of events when a germ is killed via chlorination (w12):
· disruption of cell wall barrier by reactions of chlorine with target sites on the cell surface
· release of chlorine vital cellular constituents from cell
· termination of membrane-associated functions
· termination of cellular functions within the cell
Taking this as a model for the way common germicides act, we would like to understand how this mechanism is aided by the addition of colloidal components to disinfectant solutions. Glover, et al (7) lists several reasons why surfactants are commonly used in household disinfectants. These include their ability to penetrate soil, solubilise fatty materials, wet surfaces, and their contribution to the biocidal action of the disinfectant. We may split these into two broad categories; one, encompassing the first three properties above, can be termed surface action, the other, increasing germicidal activity. The surface action, then, will have to do not with the killing of the germs but with the penetration or removal of the residues or biofilms in which they reside.
Although we have made two distinct categories above, little is actually known about how colloids, and surfactants in particular, act within cleaning solutions. Chen, et, al, showed that three surfactants commonly used in household products—SDS, Tween 20 and Triton X-100—are effective in the removal of biofilms from surfaces (3). The key to their research was in studying how biofilms are removed, whether or not the bacteria present within the film were killed. They concluded that biofilm cohesion must be governed by multiple forces, and suggested that the efficacy of surfactants to film removal might be due to “the disruption of hydrophobic interactions involved in crosslinking the biofilm matrix”(3). In addition, it was noted that the removal of a biofilm is distinct from the killing of bacteria.
Chen’s findings are important in that they elucidate one important role a surfactant can play in cleaning: the presence of organic residue or biofilms may make bacteria impervious to many disinfecting chemicals, but by adding surfactants to these chemicals the residues can be more easily penetrated or removed altogether.
Glover, et al (7), tackled the other side of the problem by studying how surfactants affect bacterial cells. They looked at several classes of surfactants—cationic, anionic, non-ionic and amphoteric—to try and determine how the surfactant structure affects its interaction with microbes. Their hypothesis stemmed from knowledge about the action of cationic surfactants. Quaternary ammonium compounds, which are extensively researched surfactants with known bactericidal effects (7), are cationic. It has been proposed that they kill cells by changing the permeability of the cell membrane. Starting from this hypothesis, Glover sought to correlate changes in the cytoplasmic membrane to the biocidal activity of surfactants.
As expected, it was found that all four types of surfactant increased the fluidity of the cell membrane by a significant amount. However, they could find no correlation between this increased fluidity and biocidal activity, which they found varied depending on the type of organism involved (7). This led them to conclude that “perturbing the fluidity of the cell membrane is not immediately responsible for cell death.” Therefore, when a surfactant used in a disinfecting solution greatly increases the bactericidal activity of the solution it is not necessarily because the surfactant is killing microbes. More likely, when the surfactant disrupts the membrane of the cell, it makes it vulnerable to the action of the accompanying disinfectant. This is in agreement with Glover’s conclusion that amphoteric and non-ionic surfactants, which showed high membrane disruption but little germicide activity, would show great adjutant ability with disinfectants.
In addition to their conclusions about how surfactants disrupt cell function, Glover et al made an important mechanistic conclusion about how the surfactant disrupts the cell membrane structure; they proposed that the increase in membrane fluidity was caused by the surfactant interfering with the packing of the phospholipid hydrocarbons that form the membrane’s lipid bilayer. This conclusion is supported by Oros, et al (13), who studied in much greater detail the structure-property relationship governing the interaction of surfactants with cells.
It is believed that both the length of the polar chain and the type of hydrophobic group affect the activity of surfactants in cell disruption (13). Oros, et al, sought to explore this more thoroughly, noting that a large range of activity could be seen in surfactants even when the hydrophobic group was the same. This would imply that the chain length was as or more important to cell interaction than the hydrophobic moiety. They compared surfactants containing polar ethylene oxide chains of varying lengths to those having none. In their study, they found that the polar chain length was the primary determinant of biocide activity. For surfactants containing no polar chain, the total length of the surfactant was smaller than the width of the lipid bilayer in the cell, and any surfactant reaching the membrane was enveloped with no apparent effect to the cell. When the chain length was too long, it interacted strongly with water outside the cell and therefore preferentially stayed in the solvent and did not enter the cell. The only surfactants capable of effectively disrupting the cell membrane were those containing ethylene oxide chains of the same length order as the membrane bilayer. These molecules were readily incorporated into the membrane, where the hydrophobic end interacted with the alkyl chains of the fatty acids while the ethylene oxide chain interacted with the phospholipid head groups (see figures below).
Figure 7a:
Schematic of cell membrane showing lipid bilayer.
Figure 7b:
Surfactants with small or no polar tail enter the lipid bilayer and are
contained without affecting membrane function.
Figure 7b:
Surfactants with an ethylene oxide tail with length on the order of the bilayer
interact
strongly with both polar and hydrophobic groups, resulting in membrane function disruption.
The papers cited above have shown how surfactants can be important components in household cleaning products, as the properties they possess due to their amphiphilic nature can clearly aid the action of disinfectants.
References
1. Behrends, Goroncy, Mohr,
and Puchstein, United Stated Patent Application: “Washing disinfectant for
hygienic and surgical hand disinfection.” Serial No. 878283, 2001.
2. Block, Seymour S., Disinfection,
Sterilization, and Preservation 5th Ed., Lippincott Williams
& Wilkins, Philadelphia, PA, 2001.
3. Chen, X. and P. S.
Stewart, “Biofilm removal caused by chemical treatments” Water
Research, 2000 Vol. 34, No. 17, pp. 4229-4233
4. Collins, Allwood, Bloomfield,
and Fox, Disinfectants: Their Use and Evaluation of Effectiveness,
Academic Press, 1981.
5. Consumer Reports,
July 2000, p. 33-37
6. Felix, Minor, and Sievert,
United States Patent Application: “Azeotrope-like compositions containing
fluoroethane.” Serial No. 901239, 2001.
7. Glover, R. E., R. R.
Smith, M. V. Jones, S. K. Jackson, C. C. Rowlands, “An EPR investigation of
surfactant action on bacterial membranes” FEMS Microbiology Letters,
1999 pp.57-62
8. E. D. Goddard, Colloids
and Surfaces, 1986, 19, 301
9. Kalyon, B D; Olgun,
U., American Journal of Infection Control, 2001, 29(2), 124-5
10. Kirk-Othmer, Encyclopedia
of Chemical Technology, 3rd Ed., Vol. 7, John Wiley & Sons,
Inc., 1997.
11. T. B. Lindman, ‘Polymer-Surfactant
Interactions: Recent Developments
in Interactions of Surfactants with Polymers and Proteins’(Chapter 5), ed. E.D.
Goddard , 1993
12. I. I. B. Magny, R. Audebert, L. Piculell, B.
Lindman, Progress in Colloid and Polymer Science, 1992, 89, 118.
13. Oros, G, T. Cserhati and E
Forgacs, “Inhibitory effect of nonionic surfactants on sunflower downy
mildew. A quantitative
structure-activity relationship study” Chemometrics and intelligent
laboratory systems, 1999 Vol 47 pp.149-156
14. Rosenberg, S., Pediatr
Infect Dis J, 2000;19(10), S114-6.
15. Spake A., Losing the
battle of the bugs: resistance to antibiotics, US News and World Report, May,
1999.8
16. Sun, Y. and Gang, S.,
Journal of Applied Polymer Science, 2002, v. 84, p. 1592-1599.10
Web References:
w1. http://www.epa.gov/oppad001/sciencepolicy.htm
w2.
http://ehpnet1.niehs.nih.gov/docs/1995/103-1/focus1.html
w3. http://www.fda.gov/cdrh/ost/reports/fy98/INFECTION.HTM
w4. http://www.messina-oilchem.com/Stimulation/Stimulation-S.html
w5. http://www.ch.kcl.ac.uk/kclchem/staff/arr/abs.htm#Ref_P97
w6. http://jan.ucc.nau.edu/~doetqp-p/courses/env440/env440_2/lectures/lec19/lec19.html
w7. “Biosecurity: Selection
and Use of Surface Disinfectants”
w8. “Clean Smarter, Not
Harder”
www.clorox.com/health/cleansmart/cleansmart5.html
w9. www.microcideinc.com/silkyfaq.htm
w10. Biosafety Program,
University of Virginia
http://keats.admin.Virginia.edu/bio/disinfectant_summary.html
w11. J. Eliz, “How does
chlorine added to drinking water kill bacteria and other harmful
organisms?” Scientific American “Ask
the Experts”
www.sciam.com/askexpert/environment/environment22/environment22.html